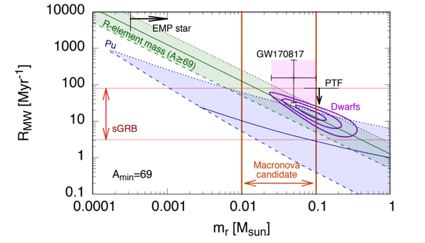
Since the dawn of humankind, people have been fascinated by the origin of gold. It appears prominently in mythologies all over the world: from the tears of the sun god Inti in the Incan culture to the cursed golden touch of king Midas in Greek mythology. This obsession resulted in a pursuit to try and artificially create gold that despite never being successful, was common enough to get its own term – alchemy. Modern physics provided us with the atomic theory, which teaches us that the distinction between different elements lies in the number of protons in their nucleus. Different types of atoms may indeed be transformed from one type to the next (as imagined by ancient followers of alchemy, although in a completely different manner), through nuclear processes such as fusion and fission.
In the astrophysical context, the formation of different elements, are dominated by different processes. For example, while most of the H and He in the Universe are relics of the big bang, slightly heavier elements are formed mainly through cosmic spallation or through nuclear fusion in the hearts of stars. Elements heavier than iron require an external source of energy to form and can therefore only occur in environments with very large densities and temperatures. About half of these elements (including gold) are dominated by the so-called rapid (or for short r) process, which requires extreme physical conditions in order to take place.
Since the fifties it has been thought that this process may take place in supernovae – the explosions that mark the end of massive stars’ lives. However, over the years, it gradually became clear that the required conditions for r-process are not readily achieved in standard supernovae. Furthermore, in the last several years, there has been growing evidence in an altogether rarer and much more exotic (but also potentially much more prolific) channel – mergers of binary neutron star systems (see figure 1).
The r-process element abundances in stars in our own Galaxy is well measured. Nonetheless it does not immediately reveal the rate of the astrophysical process behind their formation. Broadly speaking, the process may either be very common but with each event creating a meager amount of r-process
material or else the events may be rare but individually prolific. In a 2020 study, Dr. Beniamini and collaborator have shown that data from Milky Way stars can be used to constrain the rate of r-process events, as well as constrain the speed of their mixing in the interstellar medium. This is done through several techniques. First, they consider the scatter of r-process abundance (of stable r-process elements) between stars with similar iron abundances (which were therefore created in most cases, around a similar time). A greater rate of r-process events and / or speed of their mixing, leads to a smaller scatter, and vice versa. Secondly, if the speed of mixing were slow and / or the rate of enrichment high, then a fraction of the observed stars should have been formed in areas that are still greatly enriched from the latest enrichment event. This would lead to stars with huge levels of r-process enrichment which are not observed. This condition can therefore rule out combinations of sufficiently slow mixing and rapid enrichment rate. A third constraint comes from studying isotope abundance ratios as compared to their formation ratios. A greater ratio indicates a lower r-process enrichment rate and / or mixing speed. Combining all these considerations together allows to put strong constraints on the rate of r-process enrichment and the speed of mixing of those elements in the interstellar medium, which are once more consistent with neutron star mergers. Studies such as this bring us closer to deciphering the origin of gold and other r-process in the Universe and critically test our astrophysical theories.