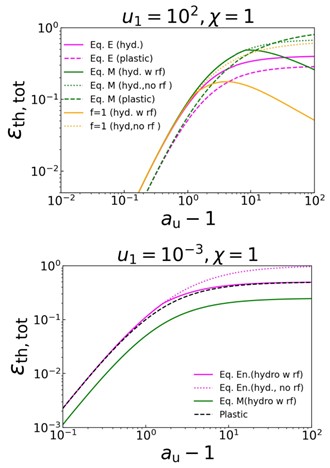
Internal shocks hydrodynamics: the collision of two cold shells in detail
Link to paper: https://academic.oup.com/mnras/article/528/1/160/7492797
Internal shocks are ubiquitous in astrophysical scenarios where outflow has matter moving at variable speeds. The faster trailing material collides with the slower leading material leading to the formation of shocks. These shocks dissipate part of the kinetic energy of the material into internal energy. A fraction of the internal energy is then channeled into electromagnetic radiation for an underlying emission mechanism. Estimation of how efficiently the shocks dissipate energy is a necessary ingredient for application in any specific astrophysical scenarios. However, most works on internal shocks make use of ballistic approximation where plastic collision of two point-like masses is assumed. But realistic estimates of thermal efficiency require solving for the shock hydrodynamics post collision. In each collision two shock fronts are generated with different shock strengths and propagation velocities and internal energy dissipation rates. In this work we undertake such an exercise and find that there can be significant differences between the efficiency estimated from the ballistic approach and the hydrodynamic approach. This is especially important when the relative velocity of the colliding masses are high. At this limit, the ballistic approach suggests very high thermal efficiency. The hydrodynamic approach suggests otherwise. When one treats the hydrodynamics we need to take into account rarefaction waves which can catch up with either of the shock fronts and halt dissipation. The rarefaction waves are triggered effectively at high relative proper speeds and lead to partially shocked shells and hence the rapid drop in thermal efficiency (see Fig 1.) This important consideration needs to be taken in any analysis of an astrophysical scenario.
The figure compares the thermal efficiency from the ballistic (dashed curves) and the hydrodynamic approach (solid curves) as a function of the relative proper speed of the colliding objects. The upper and lower panel is for collision of bodies moving at relativistic and Newtonian speeds respectively. It can be seen that at very low speed contrast the hydrodynamic efficiency is higher than the ballistic efficiency while at very high proper speed contrast the hydrodynamic efficiency is much smaller due to rarefaction waves leading to partially shocked shells.
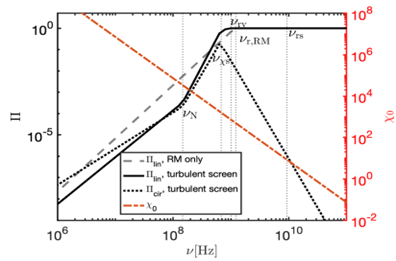
Exploring the environments of FRBs with polarization and Rotation Measure studies
Fast Radio Bursts (FRBs) are brief (typically lasting a few milliseconds), bright flashes of radio waves mostly arriving at Earth from distant galaxies. Some of the sources of FRBs have produced many observed bursts, while the majority have only been detected once so far. In one remarkable case in 2020, an FRB source was discovered in our own Galaxy. The source was identified as a known highly magnetized neutron star (called a ‘magnetar’). This has confirmed the working model, based on various less direct clues, that was held by the majority of astrophysicists studying FRBs at the time. However, the source of the Galactic FRB is much less active than some of the much more distant sources. It therefore remains unclear whether all FRBs arise from magnetars, and if so what conditions might cause a magnetar to produce such signals.
In many bursts, it is possible to measure not only the intensity of the signal but also a property known as its polarization. The very large linear polarization fraction detected in various FRBs suggests that they are produced in strongly magnetized environments (and was one of the early indications towards a magnetar origin). However, other FRBs show a much-reduced fraction of linear polarization or a large fraction of circular polarization. How should these features be understood? Can one emission site and mechanism produce such a large variety of observable features?
As radio waves propagate, they can get deflected and scattered by turbulent and ionized material (plasma) within the interstellar medium. This is a well-known phenomenon that affects radio sources. It leads to a temporal broadening of the radio signal, to modulations in the spectrum (i.e. amount of radiation received per unit frequency) and, under certain condition, it can induce also temporal variability in the observed radiation. In a work from 2022, Dr. Beniamini and collaborators have shown that in addition to the effects mentioned above, the propagation through the plasma (if the medium is sufficiently magnetized), can also affect the observed polarization. It can naturally lead to significant induced circular polarization and/or can cause depolarization of the radiation, both of which are especially pronounced at lower frequencies. This propagation effect could explain the variety of observed behaviors in FRB polarizations, without requiring the emission mechanism to vary hugely from one burst to another. Indeed, the predictions of the model align nicely with observations of various FRBs with well-studied polarization properties. However, these provide only tentative evidence. A smoking gun of such a scenario would be the measurement of a large (and strongly time variable) value of the quantity known as the ‘rotation measure’, which measured the strength of the magnetic field through the column of plasma that the radiation has propagated through
In the meantime, one of the highly active repeating sources of FRB bursts, FRB 20190520B, was monitored in great detail by some of the largest radio telescopes on Earth, for a period of over seventeen months. This monitoring revealed fluctuating polarization properties, with a reduction of polarization at lower frequencies, and a very large rotation measure. Indeed, this rotation measure was fluctuating by a huge amount and has flipped its sign from positive to negative and vice versa during the observation period. These findings matched well the results of the work mentioned above, and in 2023 were published in a paper in Science by Dr. Beniamini and collaborators (see also Open University announcement). In the case of FRB 20190520B, the analysis reveals that the turbulent plasma is likely to be highly magnetized and to reside very close to the FRB source itself. The exact origin of this plasma is still an open question, and it might indicate a magnetized wind from a neutron star surface or an outflow from a binary companion of the object producing the FRB. Either way, this type of analysis presents a new way to constrain the nature of FRB sources and their surroundings and brings us one step closer towards deciphering the mysterious nature of FRBs.
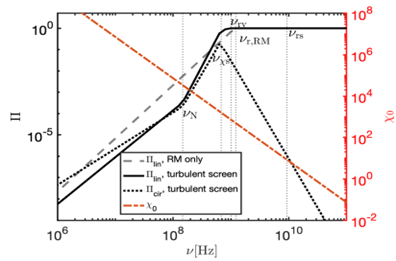
Exploring the environments of FRBs with polarization and Rotation Measure studies
Fast Radio Bursts (FRBs) are brief (typically lasting a few milliseconds), bright flashes of radio waves mostly arriving at Earth from distant galaxies. Some of the sources of FRBs have produced many observed bursts, while the majority have only been detected once so far. In one remarkable case in 2020, an FRB source was discovered in our own Galaxy. The source was identified as a known highly magnetized neutron star (called a ‘magnetar’). This has confirmed the working model, based on various less direct clues, that was held by the majority of astrophysicists studying FRBs at the time. However, the source of the Galactic FRB is much less active than some of the much more distant sources. It therefore remains unclear whether all FRBs arise from magnetars, and if so what conditions might cause a magnetar to produce such signals.
In many bursts, it is possible to measure not only the intensity of the signal but also a property known as its polarization. The very large linear polarization fraction detected in various FRBs suggests that they are produced in strongly magnetized environments (and was one of the early indications towards a magnetar origin). However, other FRBs show a much-reduced fraction of linear polarization or a large fraction of circular polarization. How should these features be understood? Can one emission site and mechanism produce such a large variety of observable features?
As radio waves propagate, they can get deflected and scattered by turbulent and ionized material (plasma) within the interstellar medium. This is a well-known phenomenon that affects radio sources. It leads to a temporal broadening of the radio signal, to modulations in the spectrum (i.e. amount of radiation received per unit frequency) and, under certain condition, it can induce also temporal variability in the observed radiation. In a work from 2022, Dr. Beniamini and collaborators have shown that in addition to the effects mentioned above, the propagation through the plasma (if the medium is sufficiently magnetized), can also affect the observed polarization. It can naturally lead to significant induced circular polarization and/or can cause depolarization of the radiation, both of which are especially pronounced at lower frequencies. This propagation effect could explain the variety of observed behaviors in FRB polarizations, without requiring the emission mechanism to vary hugely from one burst to another. Indeed, the predictions of the model align nicely with observations of various FRBs with well-studied polarization properties. However, these provide only tentative evidence. A smoking gun of such a scenario would be the measurement of a large (and strongly time variable) value of the quantity known as the ‘rotation measure’, which measured the strength of the magnetic field through the column of plasma that the radiation has propagated through
In the meantime, one of the highly active repeating sources of FRB bursts, FRB 20190520B, was monitored in great detail by some of the largest radio telescopes on Earth, for a period of over seventeen months. This monitoring revealed fluctuating polarization properties, with a reduction of polarization at lower frequencies, and a very large rotation measure. Indeed, this rotation measure was fluctuating by a huge amount and has flipped its sign from positive to negative and vice versa during the observation period. These findings matched well the results of the work mentioned above, and in 2023 were published in a paper in Science by Dr. Beniamini and collaborators (see also Open University announcement). In the case of FRB 20190520B, the analysis reveals that the turbulent plasma is likely to be highly magnetized and to reside very close to the FRB source itself. The exact origin of this plasma is still an open question, and it might indicate a magnetized wind from a neutron star surface or an outflow from a binary companion of the object producing the FRB. Either way, this type of analysis presents a new way to constrain the nature of FRB sources and their surroundings and brings us one step closer towards deciphering the mysterious nature of FRBs.
ARCO Postdoctoral positions and fellowships
The astrophysics research center of the Open University (ARCO) invites candidates to apply for 2+1 years Postdoctoral positions (extension to the third year is contingent on performance and funding). Successful candidates will pursue research in theoretical, numerical or observational astrophysics, and have full access to all the resources available at the center. We offer two types of positions: 1) A few postdoctoral researcher positions, working with one or more of the ARCO core members. 2) Exceptional candidates may be qualified for the ARCO Prize Fellowship; ARCO Prize Fellows conduct independent research as part of ARCO. Key areas of research studied by ARCO core members: Exoplanets: interior structure, thermal evolution, planet formation, exoplanet characterization (A. Vazan, H. B. Perets) High energy astrophysics: Gamma Ray Bursts, gravitational waves, compact object mergers, magnetars, fast radio bursts, r-process nucleosynthesis, tidal disruption events, supernovae and use of HEA transients as cosmological probes (J. Granot, P. Beniamini, Y. Zenati, H. B. Perets) Cosmology: Large Scale Structure formation and evolution, The Epoch of Reionization, Cosmic Dawn, the Dark Ages, redshifted 21 cm observations, intensity mapping (S. Zaroubi) General Relativity and its extensions: Quantum field theory in curved space time, black hole physics and other self-gravitating compact objects, black hole entropy and the information paradox, modified gravitational theories (M. Hadad, Y. Verbin) Dynamics: Stellar and planetary dynamics, dynamics near massive black holes. (H. B. Perets) Starting dates are expected up to the Fall of 2025. Applicants should submit a single PDF containing a cover letter, curriculum vitae, list of publications, brief (up to ~3 pages) statement of research interests and experience, and arrange for three letters of recommendation to be sent to Ms Iris Natan: irisnat@openu.ac.il

R-process Nucleosynthesis
About half of the elements in the universe heavier than iron are created via the rapid neutron capture process (the r-process). Over the recent years our understanding of the formation sites of these elements has rapidly evolved with exciting observations such as the discovery of an r-process powered electromagnetic counterpart of a binary neutron star merger detected through gravitational waves and the observation of r-process enhanced stars in some of the smallest satellite galaxies of the Milky Way. Binary neutron star mergers have emerged as a leading site for the origin of r-process elements, although many uncertainties and mysteries remain, such as the role of turbulent mixing and the observed decline in the deposition of r-process elements in the later stages of the Milky way's evolution. The next few years promise to bring big changes to our understanding of r-process formation in the Universe

Probing Cosmology and Fundamental Physics
The most extreme high-energy transient events, such as gamma-ray bursts or fast radio bursts, can be seen out to cosmological distances. This enables them to serve as powerful probes of both cosmology and fundamental physics. A prime example is testing Lorentz invariance, which is a fundamental prediction of Einstein’s theory of relativity. High-energy photons from cosmological gamma-ray bursts can test for possible violations of Lorentz invariance. In particular, they can test for a possible energy dependence of the speed of light, through a direct time of flight measurement of the arrival time of photons of different energies from the same source. An exciting application to cosmology is the use of fast radio bursts, for which the column of ionised electrons between the source and us can be measured, to study the ionization history of the Universe and the amount of baryonic matter within it. Other examples of cosmological applications are: (i) measuring the cosmological infrared background radiation through its attenuation of high-energy photons from cosmological sources, or (ii) “pair halos” that can measure the inter-galactic magnetic field strength through its bending of the trajectories of electron-positron pairs that form through the process in point (i) and thus cause a delay in the arrival time of GeV photons that arise from the pairs’ upscattering of cosmological microwave background photons.

Gamma Ray Bursts
Gamma Ray Bursts (GRBs) are the strongest cosmic explosions. They are extremely bright in hard X rays to soft gamma rays, and are associated with the collapse of massive stars (for long-duration GRBs lasting between a few seconds to minutes) or with compact star mergers (for short-duration GRBs, lasting between milliseconds and a few seconds). An ultra-relativistic jet forms at the center of the explosion and propagates out to much larger distinces where through internal dissipation it emits gamma rays known as the “prompt GRB" phase. As the jet sweeps up the external medium it drives a strong relativistic shock into it, which powers the temporally smoother and longer-lived “afterglow" emission phase. Although studied for almost 50 years, some major questions about GRBs remain unsolved. The recent discovery of a GRB counterpart to the gravitational wave signal observed from a binary neutron star merger was a spectacular milestone that has already helped address many of the open questions. Many other open questions will be similarly answered in the next few years, when more GRBs are detected as gravitational wave counterparts. Our group studies many different aspects of GRBs, including the central engines driving the explosions and the nature of the jets that they produce, their jet structure and dynamics, radiation and dissipation mechanisms in the prompt and afterglow stages of GRBs, collisionless shock physics, their polarization properties and afterglow emission.

Gravitational Wave Sources
Massive compact objects experiencing large acceleration are primary sources of gravitational waves. In particular, compact binary systems of two black holes, two neutron stars or a black hole and a neutron star are the only sources detected so far in gravitational waves. Binary neutron star systems are typically the result of two stellar collapses (i.e. supernovae). The fact that the two stars remain bound to one another after two such collapses strongly constrains the latter. As a result, the velocities of those systems, the amount of mass ejected by the collapsing star leading to the formation of the second neutron star in the system and the delay time between the formation of the binary neutron star and its eventual merger due to orbital energy losses by gravitational wave radiation, are all strongly constrained. These shed new light on the stellar evolution histories of those systems, the types of supernovae that are associated with their formation and exciting potential sources of gravitational wave radiation. Our group also studies the potential electromagnetic counterparts to such binary mergers (such as short GRBs, kilonovae and afterglows).

Fast Radio Bursts
Fast radio bursts are millisecond long radio pulses with an intrinsic rate as high as 10,000 bursts per sky per day. These mysterious bursts have been discovered as recently as 2007. The understanding of this phenomena has been dramatically evolving in the last few years, and many surprising discoveries make this an incredibly dynamic and exciting research topic. The most recent of these discoveries include the recent detection of an FRB from a magnetar in our own Galaxy, another in a globular cluster in the M81 galaxy and the discovery of periodicity in the signal of some FRBs. Understanding the conditions that lead to the formation of FRBs, their emission mechanism and the source of their potential repeatability and periodicity are among the driving theoretical questions of the moment. FRBs are also extremely promising as Cosmological probes such as the history of hydrogen reionization amongst others.

Magnetars
Magnetars are young neutron stars with the strongest magnetic fields in the Universe. They probe some of the most extreme physical conditions in nature, such as strong field gravity, huge (nuclear) densities and extreme energy densities. The evolution and decay of their ultra-strong magnetic field powers a variety of X-ray bursting activity, ranging from relatively frequent short bursts to very rare and much more energetic giant flares, which can dissipate a good part of their magnetospheric energy and eject plasma at relativistic speeds. Known magnetars are primarily Galactic, slowly spinning isolated neutron stars. Recently a Galactic magnetar was observed to emit a fast radio burst (FRB), solidifying the magnetar-FRB connection. However, despite the lack of clear direct evidence, magnetars have also been argued to play a crucial role in several other types of astrophysical transient events. In particular, a newly born rapidly rotating (with a millisecond initial spin period) magnetar at the center of an exploding massive star was suggested to be the central engine in the strongest cosmic explosions – long-duration gamma-ray bursts and super-luminous supernovae, through its powerful spin-down powered MHD wind, which taps its huge initial rotational energy, releasing it over tens of seconds to days or weeks, respectively. A broadly similar, slightly less extreme magnetar central engine may also operate in some of the more common core-collapse supernovae (CCSN). Moreover, a massive millisecond magnetar may form in an NS-NS merger and could affect the kilonova, short-ray burst, and afterglow emission.
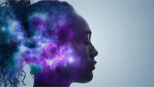
Probing Cosmology and Fundamental Physics
The most extreme high-energy transient events, such as gamma-ray bursts or fast radio bursts, can be seen out to cosmological distances. This enables them to serve as powerful probes of both cosmology and fundamental physics. A prime example is testing Lorentz invariance, which is a fundamental prediction of Einstein’s theory of relativity. High-energy photons from cosmological gamma-ray bursts can test for possible violations of Lorentz invariance. In particular, they can test for a possible energy dependence of the speed of light, through a direct time of flight measurement of the arrival time of photons of different energies from the same source. An exciting application to cosmology is the use of fast radio bursts, for which the column of ionised electrons between the source and us can be measured, to study the ionization history of the Universe and the amount of baryonic matter within it. Other examples of cosmological applications are: (i) measuring the cosmological infrared background radiation through its attenuation of high-energy photons from cosmological sources, or (ii) “pair halos” that can measure the inter-galactic magnetic field strength through its bending of the trajectories of electron-positron pairs that form through the process in point (i) and thus cause a delay in the arrival time of GeV photons that arise from the pairs’ upscattering of cosmological microwave background photons.
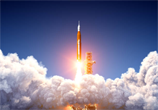
Nunc sit amet magna
Suspendisse faucibus, sem etsemper, augue quam bibendum ex, vitae facilisis lacus est vitae massa. Maecenas sit amet laoreet eros. In in tellus eget ipsum hendrerit varius sed vel nunc. Aliquam vestibulum urna nisl, quis ornare justo interdum id. Nullam sodales, urna quis consequat fermentum, est tortor imperdiet orci, sit amet porta libero urna eget ligula.
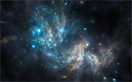
Maecenas interdum
egestas mollis. Sed accumsan est ligula, eget vestibulum eros molestie vel. Lorem ipsum dolor sit amet, consectetur adipiscing elit.
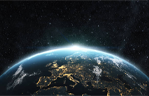
Millisecond Pu
Maecenas interdum nunc sit amet magna egestas mollis. Sed accumsan est ligula, eget vestibulum eros molestie vel. Lorem ipsum dolor sit amet, consectetur adipiscing elit.